1. Introduction
Numerous efforts have been made over the past century, and various innovation techniques have increasingly emerged and gradually established the foundation of recent significant developments. These attempts include the development of primary apparatus for placing neurosurgical probes according to Cartesian coordinate planes and cranial bony segments. Although it was exclusively applied in primates, the initial usage of stereotactic designs in the human trial to establish a minimally-invasive psychosurgical procedure with the 1st use in movement disorders, the 1st arc-centered frame, the other was publishing the 1st atlas based upon ventriculography and intracranial brain landmarks rather than bone landmarks admittedly developing a frame-based and frameless acoustic-based method for eye-tracking of the operative microscope and for tracking operative microscope, respectively was the other significant efforts in this regard. Also, with recent efforts, the spread of the prototype for frameless sonic navigation of tracking tools and instruments in human cranial surgery is mentionable that in a short time, optical digitizers were incorporated to reduce the inaccuracies from sound echoes [
1].
2. Methods and Materials/Patients
General concepts
The word “Stereotactic” is a word that originally contains two segments, the Greek word “stereos” means three-dimensional, and “tactus” as a Latin part means “touch in space” [
2]. Admittedly, the intuitive explanation of the term stereotaxy is spatial positioning, where stereo in stereotaxy means “spatial” or “three-dimensional”. “Taxis derives from the verb tattein (τάττειν) meaning “to position”[
3]. Furthermore, it is well stated that a colorful term for this surgery is “neuronavigation” So that using the stereotactic principle of co-registration of the patient with an imaging study through using images of the brain can precisely guide the surgeon to a target in the brain. However, paying attention to usual concerns about brain surgery and its complications conduces the minds to safety enhancement and, of course, smaller incisions which is the goal of this amazing technique by providing precise surgical guidance of the location of intracranial pathology [
2].
Navigation is based on targeting relative to known reference points; thus the precise definition of “fiducial”, which is especially of a point or line assumed as a fixed basis of comparison, would be significant. Fiducial is lexically originated from the Latin “fiducial”, meaning trust, which is regarded as a point of reference identified via the software package and or surgeon. Notably, the accuracy of targeting is determined using two variables, constancy of target-associated fiducials and the number of fiducials in the vicinity of the target zone. Moreover, two kinds of stereotaxy were named based on the existence of a frame and contained “frame-based stereotaxy”, which is a technique that may utilize an external frame attached to the head where fiducials play a role as bars contrived into a box or cage which settles on a frame amid imaging. In contrast, in frameless stereotaxy, reference markers, such as bone screws or stickers are employed as the fiducials directly fixed before imaging.
It is strongly believed that co-registration remains the pivotal principle of stereotaxy here, we can refer to the projects of the “animal stereotactic frame” by Horsley and Clarke in 1906, “human stereotactic frame” by Spiegel and Wycis in 1947, and the “proliferation of stereotactic frames” in the time interval of 1947- 1980 as well. Computational resources meaningfully provided frameless transformation-based stereotactic results in the 1980s [
1].
Considerations toward the frame-based stereotaxy
To begin with, considerations of frame-based stereotaxy, referring to the direct or indirect target localization method, frame placement, subsequent imaging errors, and imaging errors following the distortion, remain remarkable items. By anterior and posterior commissures (AC-PC)’s position, image-based target localization methods were performed indirectly, including standard coordinates, among which Leksell’s pallidotomy target reflects a classic paradigm and Schaltenbrand-Wahren is regarded as the most frequent adjusted map. Average AC-PC distance varies from 23 to 27 mm; otherwise, concerns about accuracy can arise in cases wider than 30 mm. Another form of the above-mentioned target localization is the direct method, where the target is visually chosen from the scan. It is noteworthy that localizing AC/PC and the midsagittal plane (MSP) is crucial in stereotactic and functional neurosurgery, human brain mapping, and medical image processing [
4].
In indirect targeting, fixed coordinates are specifically considered, including ventral intermediate nucleus (Vim) of thalamus 1-7 mm posterior, 12-17 mm lateral and 0-3 mm superior, GPi (globus pallidus internus) (2-3 mm anterior, 3-6 mm inferior, and 18-22 mm lateral) and STN (subthalamic nucleus) (3-5 mm posterior, 5-6 mm inferior and 11-14 mm lateral). Note that all aforementioned coordinates are relative to mid commissural point [
1].
3. Results
MRI image distortion
MRI image distortion is meaningfully propounded as a source of error. Non-linear spatial encoding magnetic fields and magnetic field inhomogeneities are the main contributors to distortion, often making it worst in coronal sections. Additionally, distortion severity could be determined by measuring Leksell® Coordinate frame. Besides, the aforementioned frame may introduce further distortion. Preoperatively, the process of measuring target distance using the middle cerebellar peduncle (MCP) on MRI enables the surgeon to guide targeting from the stereotaxic framed images. Importantly, not only computed tomography (CT) scan is not subject to the above-mentioned distortions but also CT/MRI fusion may lead to minimal distortion effects. Bandwidth can greatly impact contrast, for example, lower bandwidth improves white/gray contrast to a single point, and high low bandwidth can lead to a worse distortion [
1].
The frameless image-guidance
Image-guided surgery or so-called neuronavigation provides the correlation between the operative field and imaging studies. This operative technique is performed by co-registration of OR (operating room) patients with the imaging results. This technique of frameless stereotaxy, namely neuronavigation, has become a ubiquitous and pervasive tool in managing neurosurgery, including brain tumors. Understanding the spatial organization of functional regions in the nervous system enables physicians to clinically localize central nervous system (CNS) lesions, dramatically leading to enhanced precision in anatomical and functional localization in imaging studies, such as angiography, air ventriculography, and later, cross-sectional imaging modalities, including CT and MRI became available. In addition, standardizing the ability to determine the tumors and other nonneoplastic surgical targets to the finest degree and increasing accuracy empowered the current technology as far as the stereotactic coordinate system and headframe emerged through the work of Leksell and Spiegel [
5]. The logistical challenges of frame-based stereotactic navigation were dramatically reduced with the advent of frameless navigation systems [
6]. Currently, combining the data from multiple advanced imaging modalities within the singular reference frame of frameless stereotactic navigation systems enables us to predict a lesion’s location and key neighboring structural and functional regions which may be at risk during an operation [
5].
Frameless stereotactic neurosurgery needs a preoperative imaging study in which diagnostic tools such as CT scan or MRI are performed to construct a three-dimensional (3-D) anatomical map on which neuronavigation is based [
7]. Expansion of expression matrix of the following
Equation 1:
1. P2I=2ITM MTW WT3I P3I
In terms of image coverage, 2I, 2ITM stands for microscope calibration frame M, MTW stands for tracking patient and microscope, and WT3I represents patient registration, and preoperative 3D image, 3I; the index I corresponds to images 1.
Figure 1 demonstrates this procedure in detail.
Potency to quickly compute the transformations between the various coordinate systems of imaging studies, operating rooms, microscopes, and getting on for everything else is a favorite and can be considered a factual power of computational stereotaxy [
1].
Involved equipment
Without any doubt, equipment means a set of things working together as parts of an interconnecting network that contributes to creating such a complicated system. One of the main required accouterments is a localization device or so-called digitizer, such as the optical, electromagnetic, or articulated arm. Additionally, a computer providing registration algorithm as well as an effector, such as a microscope heads-up display, pointer, and monitor are required [
8].
Current co-registration strategies
Two major strategies can be used for co-registration, such as paired-point rigid transformation and surface (contour) matching. Imaging data are transferred to the surgical field to reveal the surgical instrument’s spatial position or localizer in a frameless stereotaxy. According to the surface or point-pair matching, a rigid-body-transformation algorithm is applied to measure the underlying mathematical association between the surgical working space and image volume [
1].
Before surgery, most patients scheduled to receive the frameless image-guided procedure receive MRI or CT scanning with distributed adhesive fiducial markers on their scalp. In addition, an extra fiducial marker is applied to the patient’s head as a target, just adjusted to the intracranial lesion. Preoperatively, an optical tracking system is also employed to provide 3 separate patient-to-image registration techniques, using adhesive markers, anatomical landmarks, or surface matching. Following each registration, specifying the target registration error (TRE), which stands for target registration error, was evaluated as the Euclidean distance among the world space coordinates of the target marker and image space coordinates. Regardless of imaging modality or target location, due to having a lower amount of mean TRE than the other two registration strategies, the mean application accuracy of adhesive markers is significantly higher, and it can be claimed that the registration of skin-conformal adhesive fiducial markers provides the most currently accurate non-invasive registration procedure. While the accuracy may be slightly compromised following acquired images from an earlier investigation, surface matching and anatomical landmarks provide equally accurate alternatives [
9].
4. Discussion
Error definitions
Several important definitions defined by Fitzpatrick and West should be considered. Fiducial registration error (FRE) is estimated by the root- mean square distance between corresponding fiducial points after registration; fiducial localization error (FLE) is determined as an error in locating the fiducial points. TRE is defined as the distance between the fiducial points and the corresponding points after registration.
Generally, in the first-order analysis of error in rigid point registration, it is essential to accommodate FLE and arbitrary weighting that may be inhomogeneous (varying from point to point) and anisotropic (varying with direction). Covariances are derived for both the TRE and weighted FRE and are obtained in terms of covariances of FLE, culminating in a simple implementation that embraces all compositions of weightings and anisotropy. Further, mutual independency of the fluctuations of FRE and TRE has been proved for ideal weighting, in which the weighting matrix for each fiducial equals the square root inverse of the cross-covariance of its two-space FLE. These results have been validated by both comparisons with previously published expressions and simulation [
1]. Moreover, an exhibition of simulations for randomly generated fiducial positions and FLEs displays an insignificant correlation coefficient, even correlation coefficient <0.1, in the exact case for both ideal and uniform weighting. Mainly, uniform weighting (i.e., no weighting) is utilized in commercial surgical guidance systems. Admittedly, investigation of results shows us that despite the existence the valid expressions between the covariance of FRE and the covariance of TRE for these weighting schemes, no criteria exist for the beneficence of fit of the fiducials for a certain registration that gives to first-order any information about the fluctuation of TRE from its expected value and no criteria exist to provide useful information in the detailed case. Accordingly, accomplishing the approached proceedings with extreme prudence should be considered both by the purveyors of guidance systems and by the practitioners who utilize them [
10].
According to the following
Equation 2, TRE has an approximate dependence.
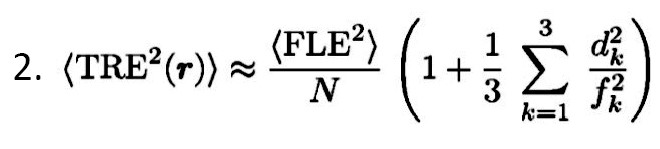
As the dependence is approached, more distance of the target occurs from the fiducial centroid, and more error is expected.
As the configuration of the fiducials became smaller, the error also enhanced. It must be accepted that FRE does not reliably identify the registration accuracy; i.e., it does not depend on fiducial
Equation 3:
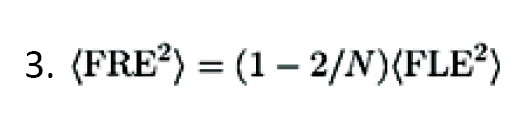
FRE remains independent of bias errors, such as digitizer camera malalignment, MRI gradient, and bent handheld probes.
Several points should be considered regarding fiducials, including the arrangement of fiducials by which the center of configuration should be adjacent to the intended region, avoiding linear fiducial configurations, avoiding distorted scalp or occipital region, utilizing fiducials as much as rationally possible, spreading out the fiducials, specified mark scalp at the patient’s fiducial region [
1].
Accuracy in phantom testing
By evolving various stereotactic neuronavigation systems in the world of neurosurgery and continuous expansion of novel designs, or instruments, concerns have been raised regarding in vitro investigations on phantoms. A phantom-based neuronavigation identifies a deformable or rigid structure within the intracranial area of the skull. Establishing phantoms is required to validate the accuracy of the procedures. Performing studies on the above-mentioned design is highly encouraged to increase procedure-related safety before the clinical recruitment of frameless, robotic, and frame-based neuronavigation designs. The aforementioned investigations aimed to estimate the targeting accuracy resulting from neuronavigation designs beyond the intracranial cavity and also to appraise the targeting errors (Entry point error [EPE], angular error [α], and target point error [TPE]). Admittedly, in the case of the availability of human-like anatomy provided by the phantom and the existence of a realistic simulation of the procedure, the value of the measured phantom is expected to be higher. Recognizing stereotactic neuronavigation phantom designs and their most pertinent features, identifying the methodologies for measuring the target point error, the entry point error, and the angular error (Alpha) [
11].
A test accuracy phantom is mainly built for use in the accuracy experiment. Fiducial markers were placed across the various regions of the phantom for registration. Also, fiducial targets along with the various bones are placed on the base of the empty cranial vault. The purpose of the accuracy experiment is to measure the baseline accuracy of the Smart Stylet system. Creating a segmented three-dimensional model of the Accuracy Phantom is the 1st step. Then the accuracy phantom was imaged in a CT scanner using a 1.25 mm slice thickness, and finally, images were segmented using Slicer software [
12].
Empirical observation, phantom, and simulation investigations have demonstrated good accuracy following an increasing number of fiducials [
1].
Stereotactic accuracy
Another usual process is to compare the precision of the stereotactic system in various studies, such as research performed by Benardete et al. which details of this study and accuracy comparison of three frameless stereotactic systems have been listed in
Table 1.
.jpg)
As well as a survey by Mascott et al., which attentively investigated the accuracy of clinical application in seven registration methods [
1]. The descriptive information related to this study has been gathered in T
able 2.
.jpg)
Furthermore, the study accomplished by Maciunas et al. demonstrates the dependence of stereotactic accuracy on image slice thickness,
Table 3 presents the data analyzed by this assay [
1].
.jpg)
This evaluation was performed toward stereotactic frames; however, the underlying principles remain the same. Besides, some important points containing no frame submillimetric, clinically meaningful is CI (range), and slice thickness plays a crucial role. Zinreich and collaborators addressed the potential shortcomings of the excellent accuracy, with an average accounting for 1-2 mm, which could be yielded in vivo, following the evaluation of the viewing wand system on a plastic skull model. Golfinos et al. obtained an accuracy of 2 mm in 82% and 92% of their patients using CT and MRI images, respectively. They demonstrated that the more accurate registration using MRI compared to CT was accompanied by a greater acquaintance rate when using MRI reconstruction in their multiple planes [
2].
To recap, it can be noted that the accuracy of frameless neuronavigation can be compromised by several factors. Due to the determining role of the slice thickness related to the scanned image on voxel resolution and the subsequent accuracy of the registration, the registration error can be enhanced following interpolation of voxel intensities in the process of image reformatting. Moreover, artifacts in the MRI or CT scan, patient motion, change in skin displacement or position of the fiducials, and faulty probe placement in the correct location of the MRI or CT scan has been considered factors that involve the accuracy of the system [
14].
Error sources
Digitizer performance, imaging data setups, and process of registration (image–OR [Operating Room] space) are well-known sources of error. Furthermore, deformation or surgical field displacement may be estimated as the sources of error as in numerous studies, the displacement is often more than 10 mm. Among imaging data setups, spatial infidelity, such as magnetic field inhomogeneities in gradient-echo-planar imaging (EPI), resolution, such as pixel/voxel size, slice thickness, and imaging study fusion, such as atlas–MRI or CT–MRI, in terms of sources of error should be highly considered [
15]. In addition, in the registration process (image–OR space), conditions, such as handedness of coordinate system, fiducial number, algorithm ambiguity, displacement or localization (digitizer and surgeon), and configuration should be considered.
Figure 2 shows a layout of this effort.
Although the source of error can be related to intraoperative MRI/CT as a result of pending cost-benefit analyses, facilitated co-registration, numerous implementations, and updated image data set [
16].
Given that actual surgical position is strongly associated with the images obtained preoperatively, and considering the patient’s brain shift that is related to the patient position, bleeding, brain edema, tumor removal, change in cerebrospinal fluid volume, cerebral blood volume, employing of mechanical ventilators, retraction or diuretics amid the surgery [
17], a progressive error in registration may occur during intraoperative navigation. In this regard, Dorward and collaborators assessed brain shifts amid open cranial surgery to measure the effect of post-imaging brain distortion on intraoperative neuronavigation, thereby acknowledging that a mean shift of 4.6mm and 6.7 mm of the cortical surface was observed following the dural opening and complete tumor resection, respectively. A significant discrepancy was observed between the shift in various tumor cases so that, for instance, deep tumor margins existed in convexity meningioma compared to gliomas, while little brain shift was represented in skull base lesions [
18].
5. Conclusion
This paper provides a review of stereotactic neuronavigation systems and their relevant features. Furthermore, this study describes various registration techniques and sources of error incorporated in stereotactic neuronavigation systems.
Remarkably, in some cases, we are facing situations, such as a tumor, including biopsy, resection of tumors harboring MET oncogene or glial tumor, epilepsy (structural and physiologic data), resection treatment of functional abnormalities (movement disorders, pain conditions) with lesion or stimulation, stereotactic implantations of neural tissue, drug transplantation, gene therapy, radiosurgery by frameless technologies, spinal fusion instrumentation, cerebrovascular abnormalities. Additionally, in other situations, including plastics, otolaryngology (ENT), ortho, and general, image guidance has great significance and has been considered an indication that utilizing neuronavigation is prominent.
The unprecedented development of image guidance has been much discussed. However, it can be acknowledged that several determinants, including updated imaging/registration, ease of use, robotic instruments, automated registration of increased accuracy, and the potential of the application to extend to other disciplines, all have been under development for image guidance.
Ethical Considerations
Compliance with ethical guidelines
This article is a review with no human or animal sample.
Funding
This research did not receive any grant from funding agencies in the public, commercial, or non-profit sectors.
Authors' contributions
All authors equally contributed to preparing this article.
Conflict of interest
The authors declared no conflict of interest.
Acknowledgment
The authors would like to offer their special thanks to Mohammad Mirahmadi Eraghi for proofreading and editing the language of the article.
References
- The Society of Neurological Surgeons. Principles of neuronavigation: Frame and frameless [Internet]. 2022 [Updated 2022 October 6]. Available fom: [Link]
- Kiss and Levy. Stereotactic surgery and frameless stereotaxy document. [Interner] 2006 [Updated 2023 January 7]. Available from: [Link]
- Grunert P Sr, Keiner D, Oertel J. Remarks upon the term stereotaxy: A linguistic and historical note. Stereotactic and Functional Neurosurgery. 2015; 93:42-9. [DOI:10.1159/000366490] [PMID]
- Liu Y, Dawant BM. Automatic localization of the anterior commissure, posterior commissure, and midsagittal plane in MRI scans using regression forests. IEEE Journal of Biomedical and Health Informatics. 2015; 19(4):1362-74. [DOI:10.1109/JBHI.2015.2428672] [PMID] [PMCID]
- Orringer DA, Golby A, Jolesz F. Neuronavigation in the surgical management of brain tumors: Current and future trends. Expert Review of Medical Devices. 2012; 9(5):491-500. [DOI:10.1586/erd.12.42] [PMID] [PMCID]
- Roberts DW, Strohbehn JW, Hatch JF, Murray W, Kettenberger H. A frameless stereotaxic integration of computerized tomographic imaging and the operating microscope. Journal of Neurosurgery. 1986; 65(4):545-9. [DOI:10.3171/jns.1986.65.4.0545] [PMID]
- Roberts DW. Stereotactic technology in tumor surgery: Concepts and principles. Journal of Neuro-Oncology. 1995; 24:281–4. [DOI:10.1007/BF01052844]
- Roessler K, Ungersboeck K, Dietrich W, Aichholzer M, Hittmeir K, Matula C, et al. Frameless stereotactic guided neurosurgery: Clinical experience with an infrared based pointer device navigation system. Acta Neurochirurgica. 1997; 139(6):551-9. [DOI:10.1007/BF02750999] [PMID]
- Woerdeman PA, Willems PW, Noordmans HJ, Tulleken CA, van der Sprenkel JW. Application accuracy in frameless image-guided neurosurgery: A comparison study of three patient-to-image registration methods. Journal of Neurosurgery. 2007; 106(6):1012-6. [DOI:10.3171/jns.2007.106.6.1012] [PMID]
- Danilchenko A, Fitzpatrick JM. General approach to first-order error prediction in rigid point registration. IEEE Transactions on Medical Imaging. 2011; 30(3):679-93. [DOI:10.1109/TMI.2011.2173637] [PMID]
- Švaco M, Stiperski I, Dlaka D, Šuligoj F, Jerbić B, Chudy D. et al. Stereotactic neuro-navigation phantom designs: A systematic review. Frontiers in Neurorobotics. 2020; 14:549603. [DOI:10.3389/fnbot.2020.549603] [PMID] [PMCID]
- Patil V, Gupta R, San José Estépar R, Lacson R, Cheung A, Wong JM, et al. Smart stylet: The development and use of a bedside external ventricular drain image-guidance system. Stereotactic and Functional Neurosurgery. 2015; 93:50-8. [DOI:10.1159/000368906] [PMID] [PMCID]
- Dorward NL, Alberti O, Velani B, Gerritsen FA, Harkness WF, Kitchen ND, et al. Postimaging brain distortion: Magnitude, correlates, and impact on neuronavigation. Journal of Neurosurgery. 1998; 88(4):656-62. [DOI:10.3171/jns.1998.88.4.0656] [PMID]
- Sharma M, Rhiew R, Deogaonkar M, Rezai A, Boulis N. Accuracy and precision of targeting using frameless stereotactic system in deep brain stimulator implantation surgery. Neurology India. 2014; 62(5):503-9. [DOI:10.4103/0028-3886.144442] [PMID]
- Peng Y, Zhang X, Li Y, Su Q, Wang S, Liu F, et al. MVPANI: A toolkit with friendly graphical user interface for multivariate pattern analysis of neuroimaging data. Frontiers in Neuroscience. 2020; 14:545. [DOI:10.3389/fnins.2020.00545] [PMID] [PMCID]
- Winkler H, Taylor KA. Marker-free dual-axis tilt series alignment. Journal of Structural Biology. 2013; 182(2):117-24. [DOI:10.1016/j.jsb.2013.02.004] [PMID] [PMCID]
- Sumanaweera TS, Adler JR Jr, Napel S, Glover GH. Characterization of spatial distortion in magnetic resonance imaging and its implications for stereotactic surgery. Neurosurgery. 1994; 35(4):696-703; discussion 703-4. [DOI:10.1227/00006123-199410000-00016] [PMID]
- Jakubovic R, Guha D, Gupta S, Lu M, Jivraj J, Standish BA, et al. High speed, high-density intraoperative 3d optical topographical imaging with efficient registration to MRI and CT for craniospinal surgical navigation. Scientific Reports; 2018; 8(1):14894. [DOI:10.1038/s41598-018-32424-z] [PMID] [PMCID]